Contents
Purification and characterisation of oligonucleotides
Introduction
Oligonucleotide synthesis involves a large number of individual reactions, which leads inevitably to the accumulation of impurities such as truncated oligonucleotides and sequences containing chemically-modified bases. These impurities must be removed after synthesis.
Chromatography is a technique for the separation of the components in a mixture by differential adsorption onto an adsorbent surface (stationary phase). The components are then eluted by a solvent or mixture of solvents (mobile phase).
In addition, it can be desirable to characterise the final product before use. A powerful method for this is mass spectrometry, often combined with chromatography.
Gel filtration
Gel filtration is the separation of the components of a mixture on the basis of molecular size, and is the simplest form of chromatography for oligonucleotide purification. Cleaved protecting groups and short truncated sequences are retained in the gel matrix while larger oligonucleotide molecules elute quickly through the gel filtration column (Figure 1). Gel filtration also removes salts from oligonucleotides that have been purified by HPLC.
While gel filtration is a very simple and useful technique, it does not remove impurities of a similar size to the desired product. It is recommended as the sole purification method only for very small (< 20 bases), unmodified oligos.
High-performance liquid chromatography (HPLC)
High-performance liquid chromatography (HPLC) is an efficient method for the analysis and purification of synthetic oligonucleotides. It can be used for the separation of oligomers of similar size and is amenable to automation. HPLC systems typically consist of the following components: an injector, pumps for delivery of solvents through the column, an interchangeable HPLC column, a column oven, a solvent mixing system, and a detector (normally UV/visible), all controlled by a PC.
Reversed-phase HPLC
Reversed-phase HPLC separates oligonucleotides and contaminants on the basis of differences in hydrophobicity. Crude oligonucleotides contain the desired product, failure sequences and chemically modified by-products, all of which will vary in hydrophobicity. For example, any oligonucleotide strands that have not been completely deprotected will be more hydrophobic than the fully deprotected product. Oligonucleotides with modifications such as fluorescent dyes are particularly suited to reversed-phase HPLC purification and reversed-phase HPLC is particularly effective in purifying short oligonucleotides (under 40 bases in length).
Figure 2 demonstrates how reversed-phase HPLC works. The mixture of oligonucleotides is injected onto a column that is made up of hydrocarbon chains (normally C8 or C18) chemically bound to a silica support of defined pore size, typically about 300 Å. Elution with aqueous ammonium acetate forces the oligonucleotide to interact strongly with the reversed-phase column. Further elution with a gradient of acetonitrile in aqueous ammonium acetate causes the individual components in the mixture to enter the mobile phase, which becomes more hydrophobic as the percentage of acetonitrile increases. Molecules with a greater degree of hydrophobicity elute more slowly, and this leads to separation of the components in the mixture.
Reversed-phase HPLC has certain limitations: the longer the oligonucleotide, the more difficult it is to separate the failure sequences of a similar length, and stable secondary structure (e.g. hairpin loops) can cause an oligonucleotide to elute as a broad peak or even a series of peaks. Placing the HPLC column in a column oven at 60 °C can overcome this problem by (temporarily) destroying secondary structure.
pH and salt concentration (ionic strength), as well as other additives and variables, can also have an effect on reversed-phase HPLC. Under reversed-phase conditions, retention is longest when the oligonucleotide is most hydrophobic. Therefore, the pH at which the analyte is least ionised will lead to the longest retention.
The pH is often adjusted using a salt. This can additionally affect retention time by forming ion pairs (longer retention) and altering the interaction with the stationary phase.
Anion-exchange HPLC
Anion-exchange HPLC separates oligonucleotides on the basis of charge differences. Each oligonucleotide in the crude mixture is a polyanion and has a particular net negative charge based on the number of phosphodiester groups in the molecule. The stationary phase on the anion-exchange column is a tertiary amine. Separation of the crude mixture is accomplished by slowly increasing the ionic strength of the mobile phase to weaken the interactions between the oligonucleotide (polyanion) and the cationic stationary phase. The longer, more highly charged oligonucleotides elute later than the shorter ones. This method is effective in separating oligonucleotides in the region of 5 to 50 bases in length from failure sequences.
Which type of HPLC should be used to purify oligos?
Reversed-phase HPLC is used routinely in the purification of oligos.
Oligos with a high degree of secondary structure (e.g. hairpin loops) give rise to multiple peaks. This secondary structure can be eliminated in the presence of a highly alkaline pH, which eliminates hydrogen-bonding interactions. Because anion-exchange HPLC can tolerate high pH, anion-exchange HPLC is a useful technique in the purification of oligos with secondary structure.
Certain oligonucleotide sequences can be predicted to give rise to secondary structure; in simple terms, oligos containing a high proportion of G and C bases (high GC content) are likely candidates for anion-exchange HPLC purification. Anion-exchange HPLC is also useful for purifying longer oligos (40–100 bases).
Hydrophobic oligonucleotides can be purified by ion-exchange HPLC by including an organic solvent in the mobile phase.
Trityl-on (DMT-on) purification
Oligonucleotides can be synthesized with the terminal 5'-DMT group attached (DMT on; trityl-on). This increases the hydrophobicity of the full-length oligonucleotide relative to all failure sequences (which will have been capped with acetic anhydride during synthesis and will not have a 5'-DMT group). Reversed-phase HPLC purification is very effective in separating the DMT-protected product from impurities. Finally, after purification, the 5'-DMT group is removed. Trityl-on purification is effective for the purification of long oligonucleotides (40 to 150 nucleotides). However, the removal of the DMT group (which requires acidic conditions) can lead to some depurination of the oligonucleotide.
Large-scale HPLC
To save materials and time, it is common to optimise HPLC gradients on an analytical (small) scale and convert them to the preparative scale using a calculator. The conversion is done by taking into account geometrical considerations such as column dimensions, the size of the particles within the column, the flow rate and the dwell volume (volume between the buffer intake point and the detector) of the HPLC system.
Fluorous affinity chromatography
Fluorous affinity chromatography exploits the concept of fluorophilicity: the high affinity of highly fluorinated organic compounds for other fluorinated compounds. Fluorous affinity chromatography requires that oligonucleotides are labelled with a fluorous tag (using a phosphoramidite with a 5'-DMT group derivatized with a perfluorinated alkyl chain; Figure 3) during solid-phase oligonucleotide synthesis. As the fluorous label is added at the end of oligonucleotide synthesis, failure sequences will not have a fluorous label. Fluorous affinity chromatography is related to trityl-on purification.
After solid-phase synthesis, the oligo is dissolved in buffer and passed through a fluorous column (containing a polymer resin to which fluorinated organic groups are bound). The labelled oligonucleotide will bind to the column strongly, while the unlabelled impurities will not interact with the column and will pass through. Subsequent washing will not release the fluorinated oligo from the column, such is the strength of the fluorophilic interaction. After all the impurities have been removed, the desired oligonucleotide is detritylated on the column (using trifluoroacetic acid), which breaks the strong interaction between the oligo and the column. The deprotected oligo can now be eluted from the column (Figure 4).
Fluorous affinity chromatography is suitable for long oligonucleotides, owing to the strength of the fluorophilic interaction. This makes it a useful method for the purification of oligos that are difficult to purify using other methods. However, the incorporation of the 5'-fluorous tag during solid-phase synthesis, which requires special 5'-fluorous phosphoramidite monomers (Figure 3, left), adds complexity and prevents the use of other 5'-modifications.
Polyacrylamide gel electrophoresis (PAGE)
Electrophoresis separates molecules based on their electrical charge and hydrodynamic properties, which are a function of chain length. PAGE purification can be used for oligos of any length, but it recommended particularly for long oligos, of around 100 bases and longer, for which other purification methods present difficulties. PAGE purification typically results in oligos of very high purity, but at the expense of yield; and can be time-consuming.
Secondary structure in oligonucleotides
The purification of certain oligonucleotides is complicated by the presence of secondary structures such as duplexes, hairpin loops (Figure 5, Figure 6) and guanine quartets (quadruplexes). Secondary structure in an oligonucleotide will influence the choice of chromatographic purification method.
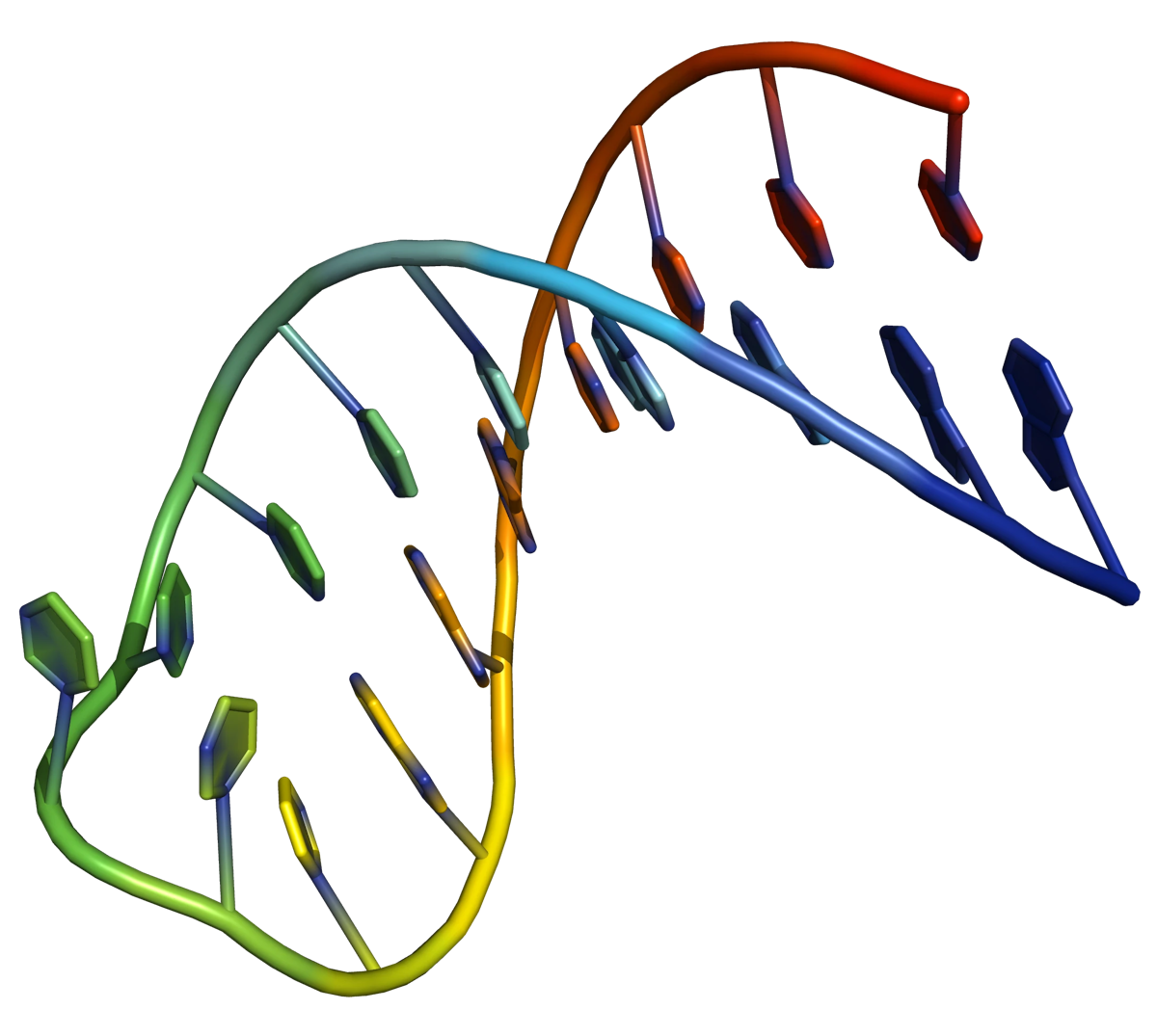
Polymer-based anion-exchange HPLC columns are useful for purifying oligonucleotides that adopt extensive secondary structure. This is because the stationary phase is stable at high pH, so HPLC purification can be carried out at pH 12. At this pH, secondary structures in the oligonucleotide are destroyed because N(3) of thymine and N(1) of guanine are deprotonated, and inter-base hydrogen bonds are destabilized. Purification at high pH is not feasible for RNA because there is a risk of 3'- to 2'-phosphate migration and cleavage of the phosphodiester backbone (see solid-phase synthesis of RNA).
Recommended methods of purification for different applications
The purification method you choose will depend on the sequence and scale of the oligo, but also on its intended use.
Application | Length | Recommended purification method(s) |
---|---|---|
PCR primer | < 20 bases | Gel filtration only, or HPLC |
PCR primer | 20–40 bases | HPLC |
PCR probe | any | HPLC |
X-ray crystallography | any | Double HPLC or PAGE |